| |
| Med Sci (Paris). 34: 116–120. doi: 10.1051/medsci/201834f120.Complement protein C5a enhances the β-amyloid-induced neuro-inflammatory response in microglia in Alzheimer’s disease Xiao-qun An,1* Wei Xi,1 Chen-yun Gu,1 and Xiao Huang2* 1MD, Department of Psychiatry, Yangpu District Mental Health Center of Shanghai, 585 Jungong Road, Shanghai(200090), China 2MD, Department of Psychological Medicine, Zhongshan Hospital, Fudan University, 180 Fenglin Road, Shanghai(200032), China |
Objective: The dysregulation of neuro-inflammation is one of the attributes of the pathogenesis of Alzheimer’s disease (AD). Over-expression of complement proteins co-localizes with neurofibrillary tangles, thereby indicating that a complement system may be involved in neuro-inflammation. Here, we report the influence of complement activation on the neuro-inflammation using a microglial cell line. Methods: first, we performed a cytotoxic assay using the microglial cells BV-2. Second, after treatment of BV-2 cells with Aβ42 and/ or C5a, the anaphylatoxin derived from C5, we determined the expression levels of the pro-inflammatory factors TNF-α, IL-1β, and IL-6. Finally, we explored whether this neuroinflammatory response was mediated by JAK/ STAT3 signaling. Results: C5a had an enhanced effect on the neural cell viability of BV-2 cells treated with Aβ42. In addition, C5a also increased the Aβ-induced neuro-inflammatory response, and these effects were blocked by the C5aR antagonist, PMX205. Finally, we demonstrated that the neuro-inflammatory responses induced by Aβ and C5a were mediated through JAK/STAT3 signaling. By blocking this pathway with an antagonist, AG490, the expression of TNF-α, IL-1β, and IL-6 was alleviated. Conclusion: The complement protein C5a could exaggerate the Aβ-induced neuroinflammatory response in microglia, and C5aR may be a potential therapeutic tool for AD treatment. Keywords: Alzheimer’s disease, β-amyloid, complement system, neuro-inflammatory response, STAT3 |
Alzheimer’s disease (AD) is one of the neurodegenerative diseases that frequently occur in elderly populations; it is characterized by cognition impairment and progressive memory loss [1]. An estimated 60 million people suffer from AD, and approximately 10 million new cases are diagnosed each year worldwide [2]. Currently, accumulation of fibrillary amyloid β (fAβ) surrounding the microglial cells and inducing the inflammatory response is believed to be a pathogenesis of AD [3]; microglial cells surrounding the senile plaques are further recruited, and neurotoxic factors (such as IL-1, TNF-α, and IL-6) are released. It aggravates the inflammatory responses, although microglial cells normally function as macrophages to help eliminate Aβ [4]. Treatment with Aβ oligomer in primary microglial cells can increase the expressions of IL-1β and TNF-α, which are significantly increased in serum and cerebrospinal fluid of AD patients [5,6]. Afterward, these inflammatory factors would feed back to the neurons and microglial cells, thereby promoting the production of other inflammatory factors. Hence, a vicious cycle is developed between the microglial cells that are activated by accumulation of Aβ and the inflammatory mediators released by microglial cells, thereby resulting in the denaturation and necrosis of neurons [7,8]. Complement system plays an important role in the identification and removal of invasive pathogenic microorganisms as one part of self-defense immune system [9,10]. Misfolded and aggregated proteins or reactive microglia found in neurodegenerative diseases can activate the complement pathways [11,12]. C5a and its receptor (C5aR) are prominently up-regulated in microglia co-localized with amyloid plaques in AD mouse models [13]. Brain tissues of AD patients revealed an increased expression of two C5 receptors, CD88, and C5L2, which was associated with abundant neurofibrillary tangles when compared with age-matched counterparts [14]. Continuously activated complement system results in excessive production of C5a and subsequently exaggerates the neuro-inflammatory response [15]. In addition, C5a enhances the injury of fibrillary amyloid β to the primary neurons [16). Hence, blocking the C5a/C5aR signaling activation axis alleviates the neuro-inflammatory alterations to AD pathologies. Both C5a-targeting vaccines and C5a receptor antagonist, PMX205, have shown improved contextual memory and reduced cerebral amyloid plaque [17, 18]. However, the underlying mechanisms by which C5a/C5aR are involved in AD pathogenesis have not been yet elucidated. Janus kinase-signal transducer and activator of transcription (JAK-STAT) has become one of the important regulatory pathways associated with AD pathogenesis [19]. STAT3, a critical nuclear transcription factor in this pathway, is involved in the neuro-inflammatory response caused by the activation of microglial cells at the onset of AD, thereby producing multiple inflammatory factors [20]. C5a was previously reported to induce STAT3 activation [21]. However, the influence of this effect in the context of AD has not been studied. Therefore, we used an Aβ oligomer to induce the in vitro inflammatory response of microglial cells and investigated the role of C5a/C5aR pathway in this process [22]. We also tried to explore the effects of C5a-induced STAT3 activation on Aβ oligomer-induced neuro-inflammatory response. |
Reagents Cell culture medium high-glucose Dulbecco’s modified eagle medium (DMEM) and fetal bovine serum (FBS) were from Gibco, USA; 0.25% trypsin and penicillin-streptomycin were from Hyclone, USA; CCK-8 kit was from Dojindo, Japan; Aβ 1-42 peptides, hexafluoroisopropanol (HFIP) and DMSO were from Sigma, USA; Trizol for extraction of total RNA and cDNA synthesis kit were from Takara, Japan; ELISA kits of TNF-α and IL-6 were from eBioscience, USA; AG490 and JNK/STAT3 specific inhibitor were from Sigma, USA; BCA protein assay kit was from Thermo, USA; rabbit anti-p-STAT3 and STAT3 polyclonal antibodies were from Cell Signaling Technology, USA; PVDF membrane and ECL kit were from Millipore, Germany. Cell culturing Microglial BV-2 cells were from the Shanghai Cell Bank of Chinese Academy of Sciences. BV-2 cells were cultured in a medium containing DMEM supplemented with 10% FBS in the 37 °C, 5% CO 2 humid atmosphere incubator. CCK-8 proliferation assay Microglial cells were inoculated onto a 96-well plate (10 5/well). After 12 h, Aβ 1-42 oligomers at different concentrations were added, and BV cells were cultured for another 24 h. The supernatant was replaced with a fresh culture medium, after which 10 μL CCK-8 reagent were added to each well and incubated for 2 h. Subsequently, OD 450 values were determined using a microplate reader. Western blot After cell lysis and centrifugation of the cell extracts at 4 °C for 15 min at 12,000 rpm, the supernatant that contains the cellular proteins was collected and used in Western-blot experiments. 20 mg of total proteins were loaded and separated through sodium dodecyl sulfonate polyacrylamide gel electrophoresis (SDS-PAGE) and then transferred onto a PVDF membrane. After incubation in blocking solution for 2 h, the PVDF membrane was probed with primary antibodies to p-STAT3 (1:1,000), STAT3 (1:500), and β-actin (1:5,000) at 4 °C overnight. After washing with TBST and probing with the relevant secondary antibodies, an ECL kit was used to reveal specific protein expression. qRT-PCR Total RNA was extracted using Trizol according to the manufacturer’s instructions. Then, 500 ng total RNA were reverse transcribed into cDNA. PCR conditions: 37 °C for 15 min, 85 °C for 5 s, and termination at 4 °C in a 10 μL system. The product of cDNA was added into the RT-PCR reaction system as template for the following procedures: initial denaturation for 30 s at 95 °C; PCR reaction for 5 s at 95 °C and 30 s at 60 °C followed by 40 cycles; and termination at 95 °C for 15 s, 60 °C for 1 min, and 95 °C for 15 s in 20 μL system. The pairs of primer sequences were: STAT-3: (Sense) 5’-TCGTGG AGCTGTTCAGTTCAGAAAC-3’, (Antisense) 5’-GGAAATTTGACCAGCAACCT-3’; IL-1β: (Sense) 5’-GGGCCTCAAAGGAAAGAATC-3’, (Antisense) 5’-TACCAGTTGG GGAACTCTGC-3’; TNF-α: (Sense) 5’-TATGGCTCAGGGTC CAACTC-3’, (Antisense) 5’-TCCCTTTGCAGAACTCAGG-3’; β-actin: (Sense) 5’-GTGCTATGTTGCTCTAGA CTTCG-3’, (Antisense) 5’-ATGCCACAGGATTCCATACC-3’. All primers were synthesized by Sangon Co., Ltd Biotech (Shanghai, PR China). The transcription level of target gene was evaluated using 2 -△△ct. Enzyme-linked immunosorbent assay (ELISA) After treatment, cell supernatants were collected to quantify the protein expression levels of IL-6 and TNF-α using ELISA detection kits according to the manufacturer’s instructions. Statistical analysis Statistical analysis was performed using the SPSS 19.0 software. In the present study, data are presented as mean ± SEM. Single-factor ANOVA was applied to compare multiple groups. t test was adopted for paired comparison. P<0.05 was set as the statistical significance. |
C5a enhances the cytotoxic effect of Ab42 on BV-2 cells Aβ 42 and C5a inhibited the cell viability of microglia. Thus, we tried to examine the effects of co-treatment with Aβ 42 and C5a on microglia. After a 24 h treatment, the cell viability of BV-2 was tested with a CCK8 assay. As shown in Figure 1, the cell number was reduced by about 50% in the Aβ 42 and C5a co-treatment group compared with Aβ 42 alone group. Cell growth was restored after adding PMX205. 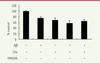 | Figure 1. Cell viability was measured by a CCK8 assay after treatment with Ab42, C5a, and PMX205 in BV-2 for 24 h. |
C5a raises the neuro-inflammatory response to Ab42 To explore the role of C5a on the inflammatory response in BV-2 cells after exposure to Aβ 42, BV-2 cells were incubated with Aβ 42, C5a, Aβ 42 plus C5a, or PMX205 for 24 h. The production of inflammatory factors, TNF-α, IL-1β, and IL-6, were analyzed. As shown in Figure 2A, the expression of these pro-inflammatory molecules increased in both Aβ 42 and C5a groups and was further up-regulated in Aβ 42 plus C5a group, whereas their expression was substantially reversed in Aβ 42 plus PMX205 group. We further measured the secreted TNF-α and IL-6 with ELISA kits. Similarly, more TNF-α and IL-6 were produced in the Aβ 42 plus C5a group than in the Aβ 42 or C5a alone group and were decreased in the Aβ 42 plus PMX205 group ( Figure 2B). 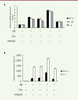 | Figure 2. Effects of C5a on the inflammatory response stimulated by Ab42. A. mRNA expression levels of TNF-α, IL-1β, and IL-6 were detected by qRT-PCR after cells were treated with Aβ42, C5a, and PMX205. B. Levels of TNF-α and IL-6 in cell culture medium were measured by ELISA. |
C5a enhances the Ab42-induced activation of STAT3 in BV-2 cells Next, we examined whether STAT3 was involved in the stimulation process of Aβ 42 and C5a in BV-2 cells. Figure 3 shows the increase in the phosphorylation of STAT3 after treatment with either Aβ 42 or C5a. This increase was further reinforced in the Aβ 42 and C5a combination group. As expected, blocking C5a with PMX205 reduced the activation of STAT3.
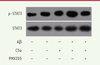 | Figure 3. Phosphorylation of STAT3 was detected in both control and treatment groups. |
The neuro-inflammatory response to Ab42 and C5a is mediated through STAT3 activation AG490, a STAT3 inhibitor, was used to investigate whether STAT3 activation mediates the neuro-inflammatory response to Aβ 42 and C5a. The production of TNF-α, IL-1β, and IL-6 was reduced after treatment with AG490 in cells exposed to Aβ 42 and C5a ( Figure 4A). Moreover, less inflammatory factors were produced when the activation of STAT3 was blocked ( Figure 4B).
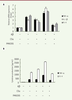 | Figure 4. Effects of the pharmacological modulation of STAT3 on inflammatory response stimulated by Ab42 and C5a. A. mRNA expression levels of TNF-α, IL-1β, and IL-6 were detected by qRT-PCR after cells were exposed to Aβ42, C5a, and AG490. B. Levels of TNF-α and IL-6 in cell culture medium were measured by ELISA. |
|
In the present study, C5a aggravated the cytotoxic effect induced by Aβ42 in BV-2 cells, consistent with previous findings, in which C5a resulted in less Aβ42-induced damage to primary neurons isolated from C5a receptor knockout (C5aR1KO) mice [16]. Moreover, C5a enhanced the neuro-inflammatory response stimulated by Aβ42 in BV-2 cells. C5aR1, one of two receptors of C5a, is expressed on the surface of primary microglia isolated from wild-type mice when compared with C5aR1 knockout mice. A previous experiment demonstrated that C5aR1+ cells surrounded Aβ plaques in AD mouse models [13], thereby suggesting that C5a/C5aR plays a role in the pathogenesis of AD. Other studies have reported that the levels of complement components increase in an age-dependent manner, thereby subsequently being able to activate more strongly complement pathways in neurons and microglia [22]. This dysregulation of complement cascade enhances the neuro-inflammatory response to fibrillary Aβ plaques in AD [23]. Hence, approaches to block C5a/C5aR activation are hypothesized to help preventing neural damage and cognitive decline. In our study, we confirmed that utilization of C5aR1 antagonist, PMX205, restored cell viability to some extent. Moreover, blocking the C5a/C5aR interaction had no influence on other complement proteins, such as C1q, C3, and C4 [13, 24], thereby suggesting that the benefit of part of the complement system is preserved. Chronic inflammatory response induced by persistently activated microglia is considered one of the major pathogenesis of AD. C5a, a pro-inflammatory factor, is actively produced after complement activation induced by Aβ deposits, which is one of the major mechanisms for microglia activation [14, 25]. The activation of microglia and the activation of the complement system further induces neuro-inflammation [23, 26]. Constant production of pro-inflammatory and complement components enhances the release of amyloid peptides [27, 28]. This self-sustaining neuro-inflammation loop between the activated microglia, complement system, and Aβ plaques ultimately results in the loss of synapses and the decline of cognitive function in AD patients [29, 30]. In the present study, we demonstrated that targeting C5a and prohibiting its receptor C5aR obviously suppressed the production of pro-inflammatory factors induced by Aβ oligomers; this finding was consistent with a previous suggestion that C5aR could be a promising therapeutic target for AD. We further demonstrated that the down-regulation of pro-inflammatory factors via treatment with C5aR antagonist was mediated through JAK/STAT3 signaling. STAT3, an important nuclear transcription factor of STAT family, mediates the signal transduction of multiple cytokines into the nucleus, thereby subsequently affecting the transcription of target genes and regulating cell function. Increasing evidence supports that the dysregulation of STAT3 is associated with the chronic inflammatory injuries in AD [31]. STAT3 is highly activated in brain tissue in AD mouse model [32]. In addition, STAT3 is involved in regulating the transcription of multiple inflammatory factors [19]. Significant decrease in the expression levels of inflammatory factors released by activated microglial cells, such as TNF-α and NO, was observed after the activation of STAT3 was blocked by AG490, a selective inhibitor of JAK signal pathway [33]. Moreover, STAT3 is involved in chronic activation of microglial cells and release of inflammatory factors induced by Aβ oligomers [34,35]. In conclusion, targeting a molecule of the complement system is an effective alternative for AD treatment. Moreover, these results may further improve our understanding of AD pathogenesis, in which chronic neuro-inflammatory response driven by both microglia and complement activation may play a pivotal role. |
Dr. Huang was financially supported by an educational grant from Zhongshan Hospital, Fudan University and Dr. An was financially supported by an grant from Yangpu District Mental Health Center of Shanghai. The authors have no potential conflict of interests.
|
1.
Prince M, Bryce R, Albanese E, Wimo A, Ribeiro W, and Ferri CP. The global prevalence of dementia: a systematic review and metaanalysis . Alzheimer‘s & Dementia. 2013;; 9: :63.–75. e2. 2.
Prince M, Wino A, Guerchet M, Ali G, Wu Y, and Prina M. World Alzheimer Report 2015. The Global Impact of Dementia: An analysis of prevalence, incidence, cost and trends . 2015. Alzheimer’s Disease International, London., 2016.
3.
Baranello
RJ
Bharani
KL
Padmaraju
V
Chopra
N
Lahiri
DK
Greig
NH
et al. Amyloid-beta protein clearance and degradation (ABCD) pathways and their role in Alzheimer‘s disease . Curr Alzheimer Res. 2015; ; 12 : :32.–46. 4.
Latta
CH
Brothers
HM
Wilcock
DM
Neuroinflammation in Alzheimer‘s disease; A source of heterogeneity and target for personalized therapy . Neuroscience. 2015; ; 302 : :103.–111. 5.
Wright
AL
Zinn
R
Hohensinn
B
Konen
LM
Beynon
SB
Tan
RP
et al. Neuroinflammation and neuronal loss precede Aβ plaque deposition in the hAPP-J20 mouse model of Alzheimer’s disease . PLoS One. 2013; ; 8 : :e59586.. 6.
Heneka
MT
Kummer
MP
Latz
E
Innate immune activation in neurodegenerative disease . Nat Rev Immunol. 2014; ; 14 : :463.–477. 7.
Heneka
MT
Carson
MJ
El Khoury
J
Landreth
GE
Brosseron
F
Feinstein
DL
et al. Neuroinflammation in Alzheimer‘s disease . The Lancet Neurology. 2015; ; 14 : :388.–405. 8.
Serrano-Pozo
A
Muzikansky
A
Gomez-Isla
T
Growdon
JH
Betensky
RA
Frosch
MP
et al. Differential relationships of reactive astrocytes and microglia to fibrillar amyloid deposits in Alzheimer disease . J Neuropathol Exp Neurol. 2013; ; 72 : :462.–471. 9.
Sarma
JV
Ward
PA
The complement system . Cell and tissue research. 2011; ; 343 : :227.–235. 10.
McGeer PL, Lee M, and McGeer EG. A review of human diseases caused or exacerbated by aberrant complement activation . Neurobiology of Aging. 2016.
11.
Crehan
H
Hardy
J
Pocock
J
Microglia, Alzheimer‘s disease, and complement . Int J Alzheimers Dis. 2012; ; 2012 : :983640.. 12.
Doens
D
Fernandez
PL
Microglia receptors and their implications in the response to amyloid beta for Alzheimer‘s disease pathogenesis . J Neuroinflammation. 2014; ; 11 : :48.. 13.
Ager
RR
Fonseca
MI
Chu
SH
Sanderson
SD
Taylor
SM
Woodruff
TM
et al. Microglial C5aR (CD88) expression correlates with amyloid-beta deposition in murine models of Alzheimer‘s disease . J Neurochem. 2010; ; 113 : :389.–401. 14.
Fonseca
MI
McGuire
SO
Counts
SE
Tenner
AJ
Complement activation fragment C5a receptors, CD88 and C5L2, are associated with neurofibrillary pathology . J Neuroinflammation. 2013; ; 10 : :25.. 15.
Girke
G
Kohl
B
Busch
C
John
T
Godkin
O
Ertel
W
et al. Tenocyte activation and regulation of complement factors in response to in vitro cell injury . Mol Immunol. 2014; ; 60 : :14.–22. 16.
Hernandez
MX
Namiranian
P
Nguyen
E
Fonseca
MI
Tenner
AJ
C5a Increases the Injury to Primary Neurons Elicited by Fibrillar Amyloid Beta . ASN Neuro. 2017; ; 9 : 1759091416687871. 17.
Landlinger
C
Oberleitner
L
Gruber
P
Noiges
B
Yatsyk
K
Santic
R
et al. Active immunization against complement factor C5a: a new therapeutic approach for Alzheimer‘s disease . J Neuroinflammation. 2015; ; 12 : :150.. 18.
Fonseca
MI
Ager
RR
Chu
SH
Yazan
O
Sanderson
SD
LaFerla
FM
et al. Treatment with a C5aR antagonist decreases pathology and enhances behavioral performance in murine models of Alzheimer‘s disease . J Immunol. 2009; ; 183 : :1375.–1383. 19.
Nicolas
CS
Amici
M
Bortolotto
ZA
Doherty
A
Csaba
Z
Fafouri
A
et al. The role of JAK-STAT signaling within the CNS . Jak-Stat. 2013; ; 2 : :e22925.. 20.
Yin L, Dai Q, Jiang P, Zhu L, Dai H, Yao Z, et al. Manganese exposure facilitates microglial JAK2-STAT3 signaling and consequent secretion of TNF-a and IL-1β to promote neuronal death . NeuroToxicology. 2017.
21.
Bai
B
Horlad
H
Saito
Y
Ohnishi
K
Fujiwara
Y
Takeya
M
et al. Role of Stat3 activation in cell-cell interaction between B-cell lymphoma and macrophages: the in vitro study . J Clin Exp Hematop. 2013; ; 53 : :127.–133. 22.
Lee
WK
Lee
SY
Choi
JE
Seok
Y
Lee
EB
Lee
HC
et al. Development of a prognosis-prediction model incorporating genetic polymorphism with pathologic stage in stage I non-small cell lung cancer: A multicenter study . Thorac Cancer. 2017; ; 8 : :251.–259. 23.
Cribbs
DH
Berchtold
NC
Perreau
V
Coleman
PD
Rogers
J
Tenner
AJ
et al. Extensive innate immune gene activation accompanies brain aging, increasing vulnerability to cognitive decline and neurodegeneration: a microarray study . J Neuroinflammation. 2012; ; 9 : :179.. 24.
Wyss-Coray
T
Rogers
J
Inflammation in Alzheimer disease-a brief review of the basic science and clinical literature . Cold Spring Harb Perspect Med. 2012; ; 2 : :a006346.. 25.
Zhou
J
Fonseca
MI
Pisalyaput
K
Tenner
AJ
Complement C3 and C4 expression in C1q sufficient and deficient mouse models of Alzheimer’s disease . J Neurochem. 2008; ; 106 : :2080.–2092. 26.
Farkas
I
Takahashi
M
Fukuda
A
Yamamoto
N
Akatsu
H
Baranyi
L
et al. Complement C5a receptor-mediated signaling may be involved in neurodegeneration in Alzheimer’s disease . J Immunol. 2003; ; 170 : :5764.–5771. 27.
McGeer
EG
McGeer
PL
Neuroinflammation in Alzheimer’s disease and mild cognitive impairment: a field in its infancy . J Alzheimers Dis. 2010; ; 19 : :355.–361. 28.
Brandenburg
LO
Konrad
M
Wruck
CJ
Koch
T
Lucius
R
Pufe
T
Functional and physical interactions between formyl-peptide-receptors and scavenger receptor MARCO and their involvement in amyloid beta 1–42-induced signal transduction in glial cells . J Neurochem. 2010; ; 113 : :749.–760. 29.
Ries
M
Sastre
M
Mechanisms of Abeta Clearance and Degradation by Glial Cells . Front Aging Neurosci. 2016; ; 8 : :160.. 30.
Stevens
B
Allen
NJ
Vazquez
LE
Howell
GR
Christopherson
KS
Nouri
N
et al. The classical complement cascade mediates CNS synapse elimination . Cell. 2007; ; 131 : :1164.–1178. 31.
Schafer
DP
Lehrman
EK
Kautzman
AG
Koyama
R
Mardinly
AR
Yamasaki
R
et al. Microglia sculpt postnatal neural circuits in an activity and complement-dependent manner . Neuron. 2012; ; 74 : :691.–705. 32.
Wan
J
Fu
AK
Ip
FC
Ng
H-K
Hugon
J
Page
G
et al. Tyk2/STAT3 signaling mediates β-amyloid-induced neuronal cell death: implications in Alzheimer’s disease . Journal of neuroscience. 2010; ; 30 : :6873.–6881. 33.
Ben Haim L, Ceyzeriat K, Carrillo-de Sauvage MA, Aubry F, Auregan G, Guillermier M, et al. The JAK/STAT3 pathway is a common inducer of astrocyte reactivity in Alzheimer’s and Huntington’s diseases . J Neurosci. 2015;; 35: :2817.–29.
34.
Huang
C
Ma
R
Sun
S
Wei
G
Fang
Y
Liu
R
et al. JAK2-STAT3 signaling pathway mediates thrombin-induced proinflammatory actions of microglia in vitro . J Neuroimmunol. 2008; ; 204 : :118.–125. 35.
Xiong
J
Wang
C
Chen
H
Hu
Y
Tian
L
Pan
J
et al. Abeta-induced microglial cell activation is inhibited by baicalin through the JAK2/STAT3 signaling pathway . Int J Neurosci. 2014; ; 124 : :609.–620. 36.
Zhang
Y
Li
S
He
H
Han
Q
Wang
B
Zhu
Y
Influence of Tanshinone IIA on apoptosis of human esophageal carcinoma Eca-109 cells and its molecular mechanism . Thorac Cancer. 2017; ; 8 : :296.–303. |