| |
| Med Sci (Paris). 34: 74–80. doi: 10.1051/medsci/201834f113.Overexpression of SLC25A15 is involved in the proliferation of cutaneous melanoma and leads to poor prognosis Shu-meng Ji1* 1Department of Dermatology, the People’s Hospital of Xintai, No.1329 of Xinfu Road, Xintai271200, Shandong, P.R. China |
Melanoma is a skin tumor with a high degree of malignancy, poor prognosis and few effective therapies. Deprivation of the arginine from cancer cells through transport inhibition and arginine depletion is a novel strategy for cancer therapy. In this study, we have investigated the effect of SLC25A15, which encodes the mitochondrial ornithine carrier 1, on melanoma progression. Using bioinformatics methods to screen the data from TCGA and GEO, we found that SLC25A15 is overexpressed in patients with melanoma and negatively related with the overall and disease-free survival rates. Knockdown the expression of SLC25A15 by siRNA could effectively inhibit the proliferation of A375 melanoma cells, as detected by CCK8 and colony formation. Furthermore, SLC25A15 siRNA was able to promote apoptosis of A375 cells, which exhibited decreased expression levels of the anti-apoptotic protein Bcl-2 while showing increased pro-apoptotic protein Bax and cleaved caspase-3. All these results suggest that the overexpression of SLC25A15 is involved in the progression of melanoma and may predict the prognosis of melanoma. This may shed new lights on the diagnosis and therapy of melanoma in the future. Keywords: A375 cells, apoptosis, cutaneous melanoma, proliferation, SLC25A15 |
Melanoma is a skin tumor with a high degree of malignancy and poor prognosis. The incidence of melanoma has increased continuously over the past few decades [1- 4]. Although surgery and adjuvant therapy can improve the prognosis of early-stage melanoma patients, the effects of these treatments are unsatisfactory for patients with tumor metastases. Chemotherapy is an important treatment for malignant melanoma; however, its therapeutic effect and indication are limited by its side effects and drug resistance properties [1, 2]. Although the use of monoclonal antibodies directed against immune checkpoints (ICP) has led to major clinical responses in patients with metastatic melanoma in the recent years, only a limited number of them have benefited of this therapeutic breakthrough. Therefore, defining the pathogenesis of melanoma and targeting key molecules involved in this process are the future directions for melanoma therapy. The metabolic differences between normal cells and tumor cells have been accepted as one of the key marks for cancer progression [5, 6]. Most of the tumor cells need additional nutrients to satisfy the elevated macromolecular biosynthesis and ATP for survival, which has inspired the development of metabolism-based therapies for cancers [7- 9]. Among the numerous candidate targets, arginine, a semi-essential amino acid, and its relationship with cancer development has been studied for many years [10- 12]. Arginine can generate many metabolites, including nitric oxide, urea, citrulline and ornithine, which are involved in the regulation of key metabolic, immunological, neurological and signaling cellular pathways and affect cell growth, proliferation and survival. Deprivation of arginine from cancer cells through transport inhibition and depletion has been a novel strategy developed for cancer therapy and has shown a promising efficacy against arginine-auxotrophic tumors [13, 14]. In addition to imported extracellular arginine, the primary intracellular arginine is de novo synthesized from citrulline through the ornithine cycle [15]. A 301 amino-acid long mitochondrial ornithine carrier 1 (ORC1) is the key transporter for cytosolic ornithine into the mitochondrial matrix in exchange for mitochondrial citrulline [16, 17]. ORC1 is encoded by the solute carrier family 25, member 15 gene (SLC25A15), which locates on chromosome 13q14 [16]. Some mutations in the SLC25A15 gene have been reported to break the ornithine cycle and be related with human hyperornithinemia, hyperammonemia, and homocitrullinuria syndrome [15, 18, 19]. However, whether the SLC25A15 gene is involved in the progression of cancers, especially melanoma, is still unknown. In this study, we first detected the differential expression of SLC25A15 in melanoma patients and normal controls. Second, we analyzed the relationship between the expression of SLC25A15 and the prognosis of melanoma patients. Finally, we investigated the roles of SLC25A15 in melanoma cell proliferation and apoptosis. Our study may shed some new lights on the diagnosis and therapy of melanoma in the future. |
Analysis of TCGA and Oncomine Data To determine the expression pattern of SLC25A15 in melanoma, the datasets in The Cancer Genome Atlas (TCGA) and Oncomine database ( https://www.oncomine.org) were used. Briefly, we used GEPIA (Gene Expression Profiling Interactive Analysis), a web-based tool to perform the differential gene analysis (Tumor: n=461; Normal: n=558) and survival analysis based on Skin Cutaneous Melanoma (SKCM) data in TCGA [ 20]. Moreover, SLC25A15 gene was queried in Oncomine and the results were filtered by selecting melanoma vs. Normal Analysis. Cell culture The cell lines used throughout this study included human cutaneous melanoma (A375, MV3) and normal human primary epidermal melanocytes (HEMa), which were all purchased at the ATCC (USA). Cells were routinely cultured in a Dulbecco’s Modified Eagle’s Medium (DMEM) (ATCC ® 30-2002™, USA) at 37 ºC in a 5 % CO2 humid atmosphere, supplemented with 100 U/mL penicillin, 0.1mg/mL streptomycin and 10% heat-inactivated fetal calf serum. Once the adherent cells reached the logarithmic phase of growth, a trypsin solution was added onto the cell layer to get single cell suspensions for further experiments. Cell transfection When cell density in the culture plate reached about 80%, they were transfected with small interfering SLC25A15 RNA (si- SLC25A15, F: 5’-GUGGAAAUACGAAUCAAGC-3’), or non-specific control si-RNA (si-con) using Lipofectamine 2000 (Invitrogen, USA) according to the manufacturer’s instructions. After a 48 h culture, the interference effect of si-RNA was evaluated by quantitative real-time reverse transcription-PCR (qRT-PCR) and the cells were used in the subsequent experiments. qRT-PCR assay After 48 h, total RNA of transfected cells were extracted by 1 mL Trizol reagent (Invitrogen, Carlsbad, CA, USA) following the manufacturer’s instructions. For cDNA synthesis, 1 µg of total RNA was used. The expression levels of SLC25A15 were assessed by qRT-PCR using SYBR Premix Ex Taq™ kit (Applied Biosystems, Foster City, CA, USA). The qRT-PCR reactions started at 95°C for 5 minutes, followed by 95 °C for 30 seconds with 40 cycles, then 60 °C for 45 seconds, finally 72 °C for 30 minutes. GAPDH (Forward, F: 5’-GGAGCGAGATCCCTCCAAAAT-3’, reverse, R: 5’-GGCTGTTGTCATAC TTCTCATGG-3’) was used as an internal control. Specific sequence primers used were SLC25A15 (F: 5’-CCTGAAGACTTACTCCCAGGT-3’, R: 5’-GCGATGTTGGCGATTAGTGC-3’). All reactions were performed in triplicate. Data analysis was performed according to the 2 -ΔΔCt method. Cell proliferation assay Forty-eight hours after transfection, cells were trypsinized and counted. 100μl cell suspension (1,000 cells/well) were then seeded into 96-well plates and cultured in 5% CO 2 incubator at 37 °C. Cell viability was tested every 24 hours. For each test, 10 μL CCK-8 (Beijing Solarbio science & technology co., ltd.) reagent were added into the wells and incubated for 1.5 h at 37 °C. Proliferation curve was plotted based on optical density (O.D.) values measured using a microplate reader at 450 nm (Bio-Rad, Hercules, CA, USA). The experiments were repeated for three times. Colony formation assay Cell suspensions (500 cells/plate) were seeded into 60 mm plate with 5 mL culture medium 48 h post- transfection. The plate was gently shaken to ensure an even distribution of the cells. After 2 weeks, cells were fixed with 4% paraformaldehyde for 30 min and stained with 0.1% crystal violet for another 30 min. Cell colonies were counted under a microscope in 5 random view fields. This experiment was repeated in triplicate. Western blot analysis Cells were harvested 48-h post-transfection. Total proteins were extracted by Radio Immunoprecipitation Assay (RIPA) Buffer (Beyotime Inc., Shanghai, China). The protein concentrations were then quantified by the BCA Protein determination method (Beyotime Inc., Shanghai, China). Proteins (20µg / lane) were separated by sodium dodecyl sulfate-polyacrylamide gel electrophoresis (SDS-PAGE) and transferred onto a polyvinylidene fluoride (PVDF) membrane. After blocking non-specific binding sites with 5% non-fat dried milk for 1 h, the membranes were incubated with primary antibodies (ORC1, 1:1000, Invitrogen; Bcl-2, 1:1000, Bax, 1:1000, caspase-3, 1:1000; β-actin, 1:5000, all from Proteintech) overnight at 4˚C. The membranes were then incubated with HRP-conjugated secondary antibody at room temperature for 1 h. Finally, the protein bands were visualized using ECL reagents (Pierce). β-actin was used as a house-keeping protein and the relative expression was calculated as a target protein / β-actin ratio. Colored bands were scanned and analyzed by QUANTITY ONE software. Statistical analysis Kaplan-Meier method and Log-rank test were used for survival analysis. The comparison of two groups was performed by Student’s t-test. When more than two groups were compared, the One-way ANOVA analysis was used. All statistical analyses were performed using the SPSS 22.0 (SPSS Inc., Chicago, IL, USA) software. The data were presented as mean ± standard deviation (SD). P<0.05 was considered statistically significant. |
Up-regulation of SLC25A15 expression in melanoma First, we analyzed the SLC25A15 mRNA expression between melanoma and normal skin tissues using the publicly available database TCGA and Oncomine. From the data of TCGA, we found that the expression of SLC25A15 was up-regulated significantly in the melanoma group as compared with the normal group ( P< 0.05, Figure 1A). Moreover, we performed an expression analysis of SLC25A15 using two datasets from Riker [ 21] and Talantov [ 22] melanoma cohorts from Oncomine. In both cohorts, the expression of SLC25A15 mRNA was significantly increased in melanoma tissues as compared to normal skin tissues ( Figure 1B–C). Second, the relationship of SLC25A15 overexpression with prognosis in all 456 melanoma patients was also analyzed. Based on the median value of SLC25A15 expression, patients were divided into two groups with high (n = 229) and low (n = 227) SLC25A15 levels, respectively. The results showed that patients with high SLC25A15 mRNA expression had shorter overall and disease-free survival times than those with low SLC25A15 (both P<0.01, Figure 2A–B). These results suggested that overexpressed SLC25A15 is correlated with a poor prognosis in melanoma patients.
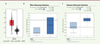 | Figure 1.Overexpression of SLC25A15 in melanoma patients from TCGA and Oncomine databases. A. Data from TCGA database. Tumor group: red column; non-tumor group: gray column. B-C: data from Oncomine database (Riker [ 21] and Talantov [ 22]) are plotted. The boxes represent data from the 25 th to 75 th percentiles. The horizontal lines are the medians. The whiskers represent the 10 th and 90 th percentiles, respectively. |
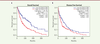 | Figure 2.
Poor prognosis of SLC25A15 overexpression in melanoma patients from TCGA database. A. SLC25A15 high expression is associated with a poor overall survival in melanoma patients. B. SLC25A15 high expression is associated with a poor disease-free survival in melanoma patients. Kaplan–Meier plots of survival were generated by the software from Gepia (http://gepia.cancer-pku.cn
) using the data from TCGA. |
SLC25A15 is overexpressed in cutaneous melanoma cells To further characterize the expression of SLC25A15 in melanoma, we analyzed its mRNA level in normal melanocytes and some cutaneous melanoma cell lines, including A375, MV3, WM-115 and SK-MEL-3, using qRT-PCR. As shown in
Figure 3
, the expression of SLC25A15 in cells from the melanoma cell lines was higher than in the HEMa normal melanocytes (all P<0.01). These results obtained in melanoma cell lines were consistent with the conclusion draw from database analyses. Moreover, the expression of SLC25A15 in A375 cells was the highest among all tested melanoma cell lines. Therefore, in the next in vitro experiments, A375 cells were used.
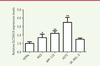 | Figure 3.
Overexpression of SLC25A15 in melanoma cell lines by q-PCR. Data are represented as means ± S.D. of three independent experiments. * P<0.05, ** P<0.01 compared with the HEMa group. |
Decreased proliferation of SLC25A15-knocked down A375 cells For better evaluating the effect of SLC25A15 on melanoma progression, a SLC25A15 siRNA (si- SLC25A15) was transfected into A375 cells. Forty-eight hours later, the expression of SLC25A15 RNA and protein in A375 cells was detected by qRT-PCR and western blot to assess the effect of si- SLC25A15. Our results showed that si-SLC25A15 could significantly reduce the RNA and protein expression levels of SLC25A15 in A375 cells, and the knockdown efficiencies were over 70% (
Figure 4A–C
, P<0.01). Then we studied the effect of SLC25A15 on cutaneous melanoma cells by using the siRNA. Firstly, cell proliferation activity was examined using a CCK-8 assay. Silencing of SLC25A15 remarkably inhibited the viability of A375 cells as compared to the control at 72 h ( P<0.05) and 96 h (
Figure 4D
, P<0.01). Subsequently, a colony formation assay was also performed. We found that A375 cells transfected with SLC25A15 siRNA formed fewer clones than the control (
Figure 4E–F, P<0.01). These data suggest that SLC25A15 may play a promoting role in melanoma proliferation.
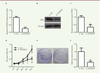 | Figure 4.
SLC25A15 knockdown inhibits the proliferation of A375 cells. A. Relative expression levels of SLC25A15 in A375 cells after treatment with small interfering RNA (q-PCR). B-C. Relative protein expression levels of ORC1 in A375 cells after treatment with small interfering RNA (Western blot). D. SLC25A15 knockdown decreases the viability of A375 cells as shown by a CCK8 assay. E-F. SLC25A15 knockdown inhibits colony formation of A375 cells in a colony formation assay. All values are presented as means ± S.D. * P<0.05, ** P<0.01 compared with si-con group. n=5 for each group. si-con, scramble control siRNA. |
Decreased expression of SLC25A15 promotes the apoptosis of A375 cells Given the fact that the apoptosis plays an important role in controling cell proliferation, we then tested the expression of apoptosis-related proteins in A375 cells with or without siRNA treatment. The western blot results showed that the expression levels of the anti-apoptotic protein Bcl-2 was decreased; by contrast, the pro-apoptotic protein Bax and cleaved caspase-3 levels were increased ( Figure 5A–B, all P<0.01). These results indicate that SLC25A15 could inhibit the apoptosis of A375 cells.
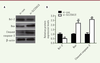 | Figure 5.
Silencing SLC25A15 promotes the apoptosis of A375 cells. (A) Representative images of the expression of Bcl-2, Bax and cleaved caspase-3 by western blot analysis. (B) Quantification of Bcl-2, Bax and caspase-3 protein expression. All values are presented as means ± S.D. ** P<0.01 compared with si-con group. n=5 for each group. si-con, scramble control siRNA. |
|
To our knowledge, there is no study showing the effect of SLC25A15 in melanoma. In this study, for the first time, we identified an overexpression of SLC25A15 in melanoma, which was negatively related with the survival rate of patients. Moreover, we also found an up-regulation of SLC25A15 in some melanoma cells compared with the normal melanocytes. Importantly, SLC25A15 knockdown inhibited the A375 cell proliferation and promoted their apoptosis. Arginine is a crucial candidate for various molecular pathways and thus the accessibility of arginine can regulate key metabolic, neurological and immunological pathways of the cells [23]. Because of the rapid growth of cancer cells, their demand for arginine is very high, which causes a metabolic reprogramming in some cancers [24- 26]. SLC25A15 encoded ORC1 is important for the endogenous arginine biosynthesis, and the mutation of SLC25A15 could lead to the unbalance of arginine metabolism and the appearance of the HHH syndrome [15]. In our study, we found that the overexpression of SLC25A15 in melanoma is necessary for the proliferation of A375 melanoma cells. Previous reports have indicated that several types of tumors, including melanoma, have abnormalities in arginine metabolism-related enzymes, particularly arginine-succinate synthase (ASS), and depend primarily on extracellular arginine to support necessary biological processes [27- 29]. The appearance of SLC25A15 overexpression in melanoma might accelerate the endogenous synthesis of arginine to compensate for the arginine auxotrophy. Moreover, we also found the overexpression is negatively related with the survival rate of patients. These results suggest that SLC25A15 is a promising novel diagnostic marker and therapeutic target for human skin melanoma in the future. Early findings suggest that exogenous arginine deprivation can induce apoptosis and cause some the death of cells from cancer cell lines, including breast cancer, prostate cancer, mesothelioma, and melanoma cell lines [30- 33]. Although the signaling pathway responsible for this apoptosis is still unknown, it is believed that the apoptosis caused by arginine elimination could be activated through caspase-dependent and/or independent pathways [11]. In the present study, we also showed that the content in the anti-apoptosis protein Bcl-2 decreased, while those of the pro-apoptosis protein Bax and cleaved caspase-3 increased after SLC25A15 knockdown. Our findings are consistent with previous reports and suggest that the targeting of SLC25A15 may be a novel anti-melanoma method through promoting apoptosis. In conclusion, our study firstly reported that the overexpression of SLC25A15 is an important biomarker of melanoma development. The inhibition of the SLC25A15 expression could effectively block the proliferation of A375 melanoma cells via promoting apoptosis. It might shed new lights on the diagnosis and therapy of cutaneous melanoma. However, our study is a preliminary study that needs more works to be done to clarify the roles and mechanisms of SLC25A15 in melanoma. |
The authors state that there are no competing interests in this work.
|
1.
Marconcini
R
Spagnolo
F
Stucci
LS
et al. Current status and perspectives in immunotherapy for metastatic melanoma . Oncotarget. 2018; ; 9 : :12452.–12470. 2.
Yang
J
Manson
DK
Marr
BP
Carvajal
RD
Treatment of uveal melanoma: where are we now? . Ther Adv Med Oncol. 2018; ; 10 : :1758834018757175.. 3.
El-Kenawy AEM, Constantin C, Hassan SMA, et al. Nanomedicine in Melanoma: Current Trends and Future Perspectives . In: Ward WH, Farma JM, eds. Cutaneous Melanoma: Etiology and Therapy . Brisbane (AU): 2017.
4.
Matthews NH, Li WQ, Qureshi AA, et al. Epidemiology of Melanoma . In: Ward WH, Farma JM, eds. Cutaneous Melanoma: Etiology and Therapy . Brisbane (AU): 2017.
5.
Jia D, Park JH, Jung KH, et al. Elucidating the Metabolic Plasticity of Cancer: Mitochondrial Reprogramming and Hybrid Metabolic States . Cells. 2018; :7..
6.
Min
HY
Lee
HY
Oncogene-Driven Metabolic Alterations in Cancer . Biomol Ther (Seoul). 2018; ; 26 : :45.–56. 7.
Kouidhi S, Ben Ayed F, Benammar Elgaaied A. Targeting Tumor Metabolism: A New Challenge to Improve Immunotherapy . Front Immunol. 2018;; 9 : :353..
8.
Choi
YK
Park
KG
Targeting Glutamine Metabolism for Cancer Treatment . Biomol Ther (Seoul). 2018; ; 26 : :19.–28. 9.
Luengo
A
Gui
DY
Vander Heiden
MG
Targeting Metabolism for Cancer Therapy . Cell Chem Biol. 2017; ; 24 : :1161.–1180. 10.
Morris
CR
Hamilton-Reeves
J
Martindale
RG
et al. Acquired Amino Acid Deficiencies: A Focus on Arginine and Glutamine . Nutr Clin Pract. 2017; ; 32 : :30S.–47S. 11.
Patil
MD
Bhaumik
J
Babykutty
S
et al. Arginine dependence of tumor cells: targeting a chink in cancer‘s armor . Oncogene. 2016; ; 35 : :4957.–4972. 12.
Han
RZ
Xu
GC
Dong
JJ
Ni
Y
Arginine deiminase: recent advances in discovery, crystal structure, and protein engineering for improved properties as an anti-tumor drug . Appl Microbiol Biotechnol. 2016; ; 100 : :4747.–4760. 13.
Xiong
L
Teng
JL
Botelho
MG
et al. Arginine Metabolism in Bacterial Pathogenesis and Cancer Therapy . Int J Mol Sci. 2016; ; 17 : :363.. 14.
Qiu
F
Huang
J
Sui
M
Targeting arginine metabolism pathway to treat arginine-dependent cancers . Cancer Lett. 2015; ; 364 : :1.–7. 15.
Ersoy Tunali N, Marobbio CM, Tiryakioglu NO, et al. A novel mutation in the SLC25A15 gene in a Turkish patient with HHH syndrome: functional analysis of the mutant protein . Mol Genet Metab. 2014;; 112 : :25.–9.
16.
Palmieri
F.
The mitochondrial transporter family SLC25: identification, properties and physiopathology . Mol Aspects Med. 2013; ; 34 : :465.–484. 17.
Palmieri
F.
Mitochondrial transporters of the SLC25 family and associated diseases: a review . J Inherit Metab Dis. 2014; ; 37 : :565.–575. 18.
Marobbio
CM
Punzi
G
Pierri
CL
et al. Pathogenic potential of SLC25A15 mutations assessed by transport assays and complementation of Saccharomyces cerevisiae ORT1 null mutant . Mol Genet Metab. 2015; ; 115 : :27.–32. 19.
Salvi
S
Dionisi-Vici
C
Bertini
E
et al. Seven novel mutations in the ORNT1 gene (SLC25A15) in patients with hyperornithinemia, hyperammonemia, and homocitrullinuria syndrome . Hum Mutat. 2001; ; 18 : :460.. 20.
Tang
Z
Li
C
Kang
B
et al. GEPIA: a web server for cancer and normal gene expression profiling and interactive analyses . Nucleic Acids Res. 2017; ; 45 : :W98.–W102. 21.
Ren
S
Liu
S
Howell
P, Jr
et al. The impact of genomics in understanding human melanoma progression and metastasis . Cancer Control. 2008; ; 15 : :202.–215. 22.
Talantov
D
Mazumder
A
Yu
JX
et al. Novel genes associated with malignant melanoma but not benign melanocytic lesions . Clin Cancer Res. 2005; ; 11 : :7234.–7242. 23.
Wu
G.
Functional amino acids in growth, reproduction, and health . Adv Nutr. 2010; ; 1 : :31.–37. 24.
Cantor
JR
Sabatini
DM
Cancer cell metabolism: one hallmark, many faces . Cancer Discov. 2012; ; 2 : :881.–898. 25.
Ferreira
LM
Hebrant
A
Dumont
JE
Metabolic reprogramming of the tumor . Oncogene. 2012; ; 31 : :3999.–4011. 26.
Dillon
BJ
Prieto
VG
Curley
SA
et al. Incidence and distribution of argininosuccinate synthetase deficiency in human cancers: a method for identifying cancers sensitive to arginine deprivation . Cancer. 2004; ; 100 : :826.–833. 27.
Savaraj
N
Wu
C
Li
YY
et al. Targeting argininosuccinate synthetase negative melanomas using combination of arginine degrading enzyme and cisplatin . Oncotarget. 2015; ; 6 : :6295.–6309. 28.
Long
Y
Tsai
WB
Wangpaichitr
M
et al. Arginine deiminase resistance in melanoma cells is associated with metabolic reprogramming, glucose dependence, and glutamine addiction . Mol Cancer Ther. 2013; ; 12 : :2581.–2590. 29.
Feun
LG
Marini
A
Walker
G
et al. Negative argininosuccinate synthetase expression in melanoma tumours may predict clinical benefit from arginine-depleting therapy with pegylated arginine deiminase . Br J Cancer. 2012; ; 106 : :1481.–1485. 30.
Karunakaran
S
Ramachandran
S
Coothankandaswamy
V
et al. SLC6A14 (ATB0,+) protein, a highly concentrative and broad specific amino acid transporter, is a novel and effective drug target for treatment of estrogen receptor-positive breast cancer . J Biol Chem. 2011; ; 286 : :31830.–31838. 31.
Kim
RH
Coates
JM
Bowles
TL
et al. Arginine deiminase as a novel therapy for prostate cancer induces autophagy and caspase-independent apoptosis . Cancer Res. 2009; ; 69 : :700.–708. 32.
Wangpaichitr
M
Wu
C
Bigford
G
et al. Combination of arginine deprivation with TRAIL treatment as a targeted-therapy for mesothelioma . Anticancer Res. 2014; ; 34 : :6991.–6999. 33.
Savaraj
N
You
M
Wu
C
et al. Arginine deprivation, autophagy, apoptosis (AAA) for the treatment of melanoma . Curr Mol Med. 2010; ; 10 : :405.–412. |